Let’s Talk! Interdisciplinary dialogues help to operationalize Socio-Ecological Systems Resilience and Sustainability
Mengyu Liang (Amber) ·From a physical geographer's perspective, the social aspects of understanding the Earth's environment have always been around me. However, how humans interact with their environment is more treated as noises or jittering that happen in a short time scale around the main geomorphological trends that are hundreds of million years long; we talk about mountain building through plate collisions, the folds and faults of rocks, the erosion from water, wind, and ice, and only mention human's interplay in the long process when discussing relatively "ephemeral" land covers and land-use conversions, such as mining and farming. This view, however, underestimates these "ephemeral" human phenomena's impact on the Earth system, especially when climate changes become the central concerns of our society.
A striking example provided by our first guest lecturer demonstrates the impactful role of human and social organization in natural systems. Dr. Mitch Pavao-Zuckerman who is an urban ecologist and an assistant professor at the Department of Environmental Science & Technology shared some of his insight on the Biosphere 2 project from being a pos-doc at the University of Arizona. He said there were many failures on the ecological sides of the project associated with CO2 being lower than originally calculated thus leading to reduced plant photosynthesis and other uncalculated effects. He also identified another "uncalculated" component related to the people or the crew; their dynamics, hierarchy, and organization added another layer of uncertainty to the outcome of the whole project. This goes to show that as much as we know about the functions of the natural system, it is still extremely difficult to recreate the Earth system, where human and nature interplay and have inseparable relationships with each other.
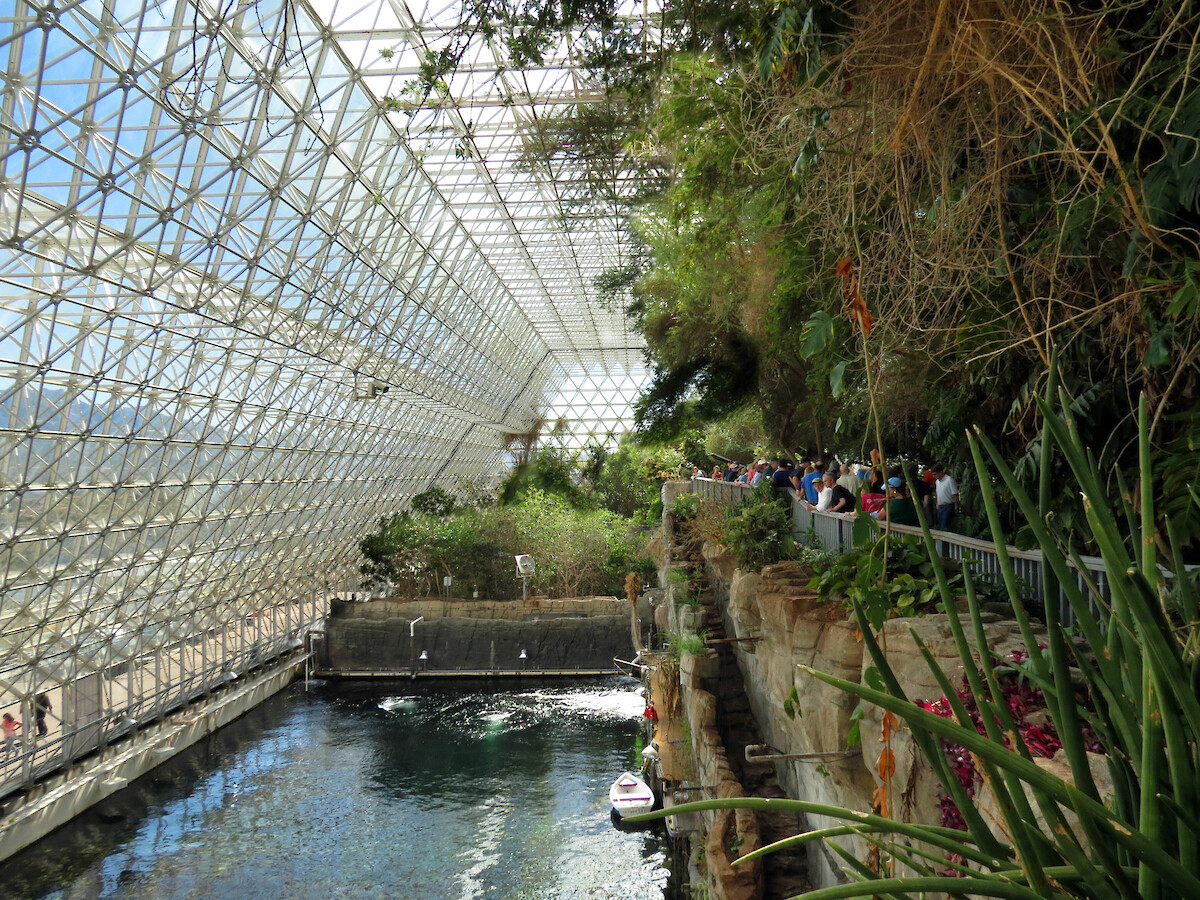
The topic of system resilience is another good demonstration that makes me think about how social and ecological systems intertwine in a deeper way. Resilience is a concept that has been defined differently in different fields. For engineers, resilience concerns the stability of a system, so how fast a system returns to equilibrium (Holling, 1996). For ecologists, resilience is more related to a system's buffer capacity, and how much shock the system can withstand to maintain normal functions (Holling et al., 1995). While the engineering perspective's focus does not realistically capture the nature of the evolving ecological system, the pure ecological perspective also does not adapt quickly enough to the Earth system's variations under global climate change. The rising of the coupled social-ecological perspective of examining resilience, hence, provides a more dynamic way of addressing integrated system feedback and cross-scale interactions when every chain of the Earth system is altered by climate change (Folke, 2006). Under this view, resilience becomes an intrinsic character of the system that highlights the interlinkages of socioeconomic activities and ecological functions.
Although this perspective is a more realistic and suitable metaphor for depicting the Earth system under climate change, Dr. Mitch Pavao-Zuckerman also recognizes the difficulty in its operationalization. The question now becomes, yes, we know the two aspects of categorizing systems, and they overlap, but how to implement such transdisciplinary studies to examine the adaptive capacity, transformability of social-ecological system resilience, or, how to get them to talk to each other? Dr. Mitch Pavao-Zuckerman pointed to the role of scales in the discussion of this issue, and indicated the examination of "specific resilience", in contrast to "general resilience", is perhaps easier through small-scale studies of a subsystem. One of the examples he described is socio-hydrology, where the more specific question is, "are people just responsive to the hydrological cycle? Or are they driving the cycle", is asked. Or, as would be approached by critical geographers, how people use the water cycle can be studied by examining how the hydro cycle manifests in urban systems.
Our course instructor, Dr. Bill Dennison, asked a thought-provoking question followed with a great answer, which helped shed light on the operationalization of studying social-ecological resilience. He asked what about water that made it such a great point of penetration for this difficult discussion of operational social-ecological resilience? And he indicated that many people experience climate change through water dynamic alteration, such as the prevalence of drought, and water level change. The example of water acts as a good reminder that real-life needs could be great inspirations or drive the operational study of specific social-ecological resilience. (Striking scientific simulations examples of sea level trend in Figure 2 below). But what about broader or general operationalization? I think the indicator-based approach demonstrated by Dr. Xin Zhang through her discussion on the Sustainable Agriculture Matrix (SAM) project helped to demonstrate.
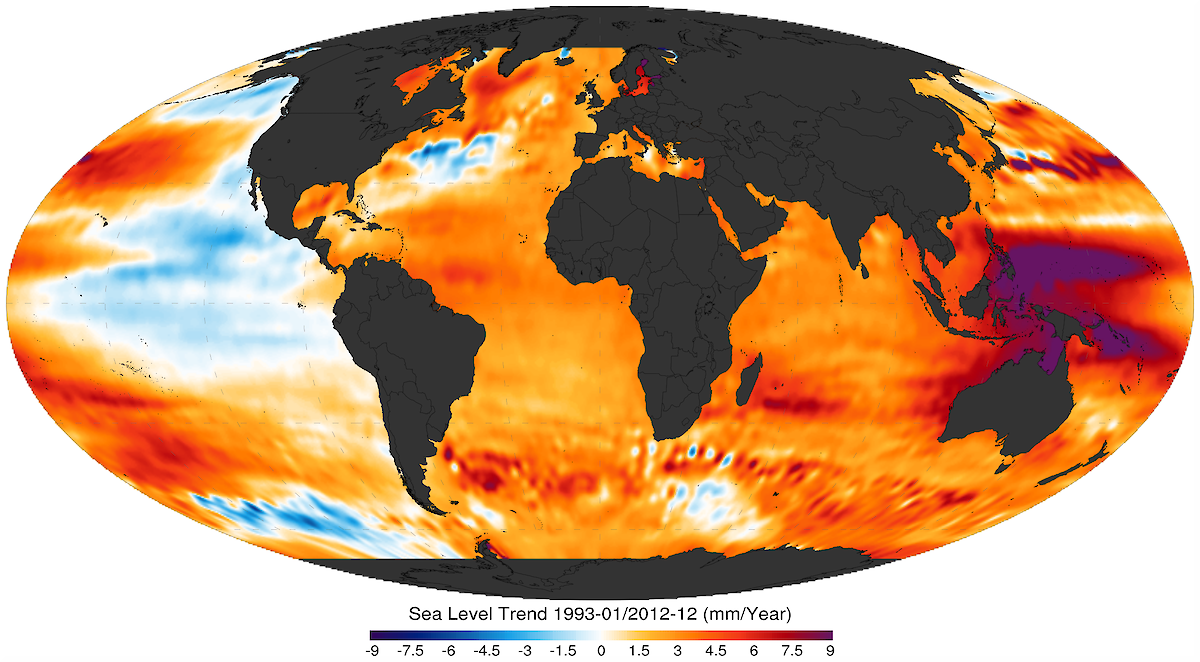
Before discussing how SAM put the social-ecological perspective in operation, Dr. Xin Zhang, a sustainable development ecologist and assistant professor at the Appalachian Laboratory of University of Maryland Center for Environment Science (UMCES), also emphasized the importance of social and ecological perspectives concerning sustainability. While sustainability is considered a normative state of a system where variables return to the original standard after disturbance, if discussed from a resource management point of view, sustainable development is double-bonded and resembles a doughnut (Figure 3) where the upper limits are the environmental ceiling and the lower boundary is the socio-economic foundation that fulfills human needs (Raworth, 2012).
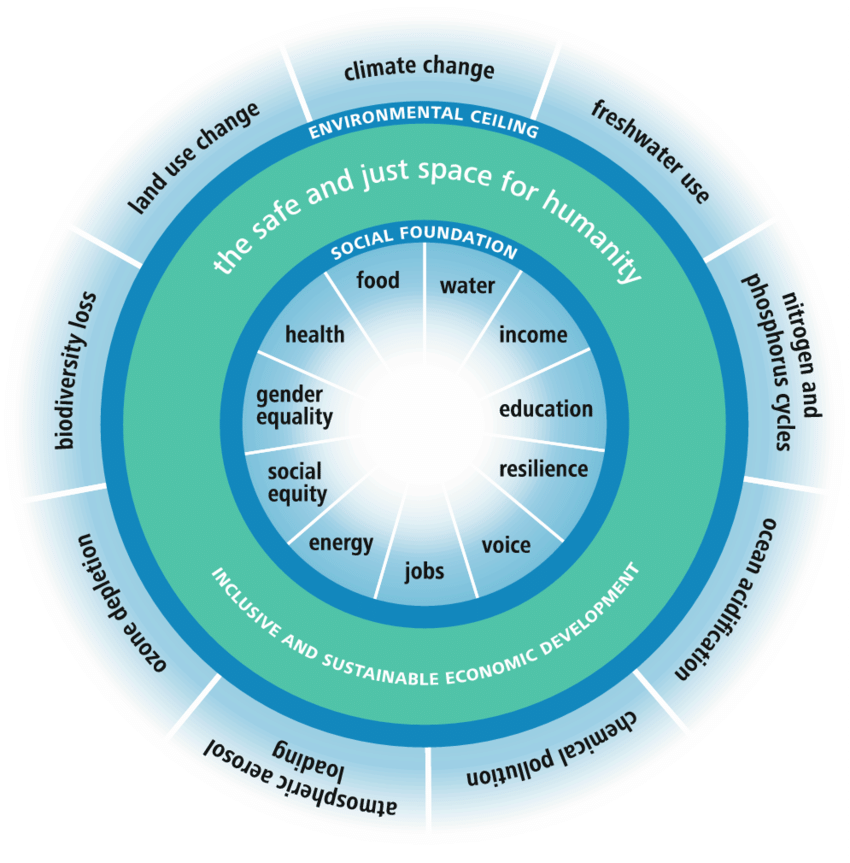
In response to the Sustainable Development Goals (SDG) initiated during the United Nations (UN) Rio+20 conference in 2012 (Figure 4), Dr. Xin Zhang and her collaborators focused on sustainable agriculture (SA) development by using collective metrics to evaluate the specifics of SA from social, economic and environmental aspects. She indicated that they have identified 100+ natural and social indicators to assess and investigate the drivers of agriculture sustainability more transparently and consistently. Data and knowledge from disciplines like remote sensing, forest science, food science, sociology, ecology, and politics all play a role through these indicators (Figure 5). Although tradeoffs are quite common at this stage of sustainable development, she indicated this kind of all-around approach helps to track performance and monitor any unintended consequences. Dr. Xin Zhang and her team's SAM project is a great example of how to put the social-ecological approach in operations in the study of sustainability.
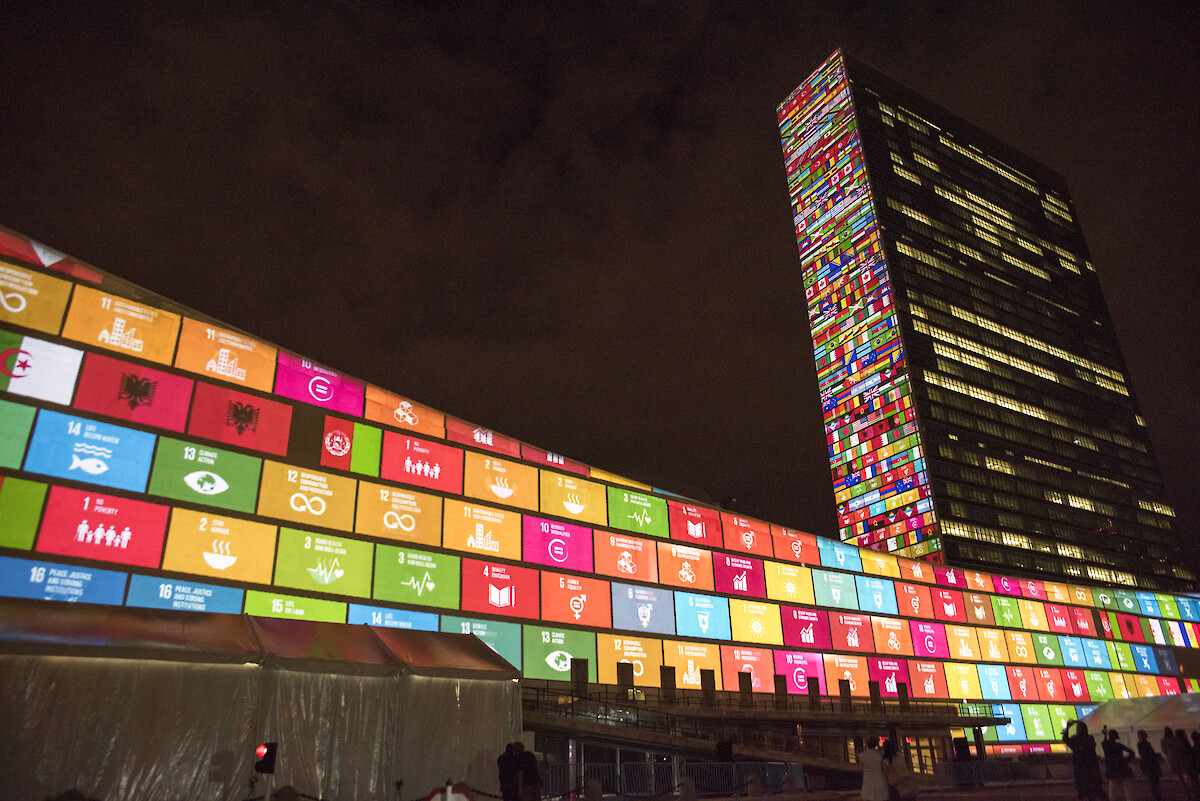
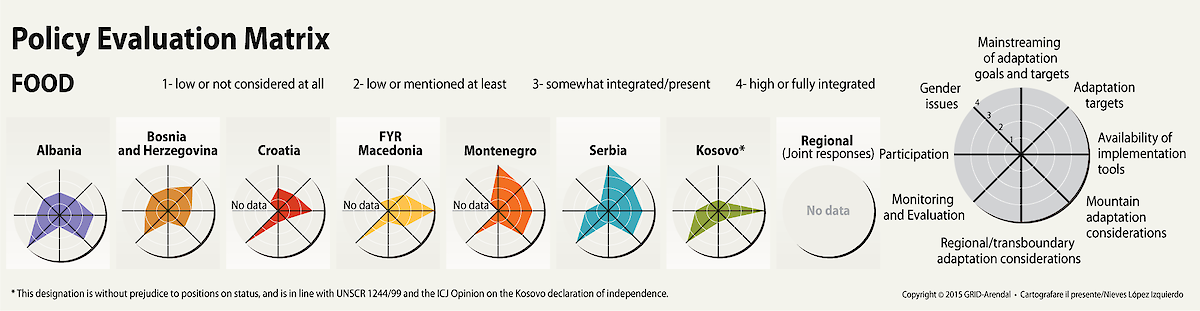
Last notion on operationalizing the social-ecological perspective to examine system resilience and sustainability arises from the student discussion. One student in class asked about the role diversity plays in system resilience. Related to diversity in species, resilience is key to biodiversity conservation, and diversity itself enhances resilience. Ecosystems that provide higher degrees of diversity/ or complexity could reduce vulnerability and increase the buffer capacity of both the social and ecological systems (Adger, 2000). From the social aspect, more diverse participants stimulate information flow which could lead to more options and more mature consideration being raised. This, to me, also highlights the importance of interdisciplinary research. Because, as we reflected at the beginning of the class, science is also a cultural model which is not always objective and neutral as we would like to think, but the joining of diverse experts from both social and natural science domains, perhaps could increase accountability and invigorate problem-solving (Nature, 2018) (Figure 6).
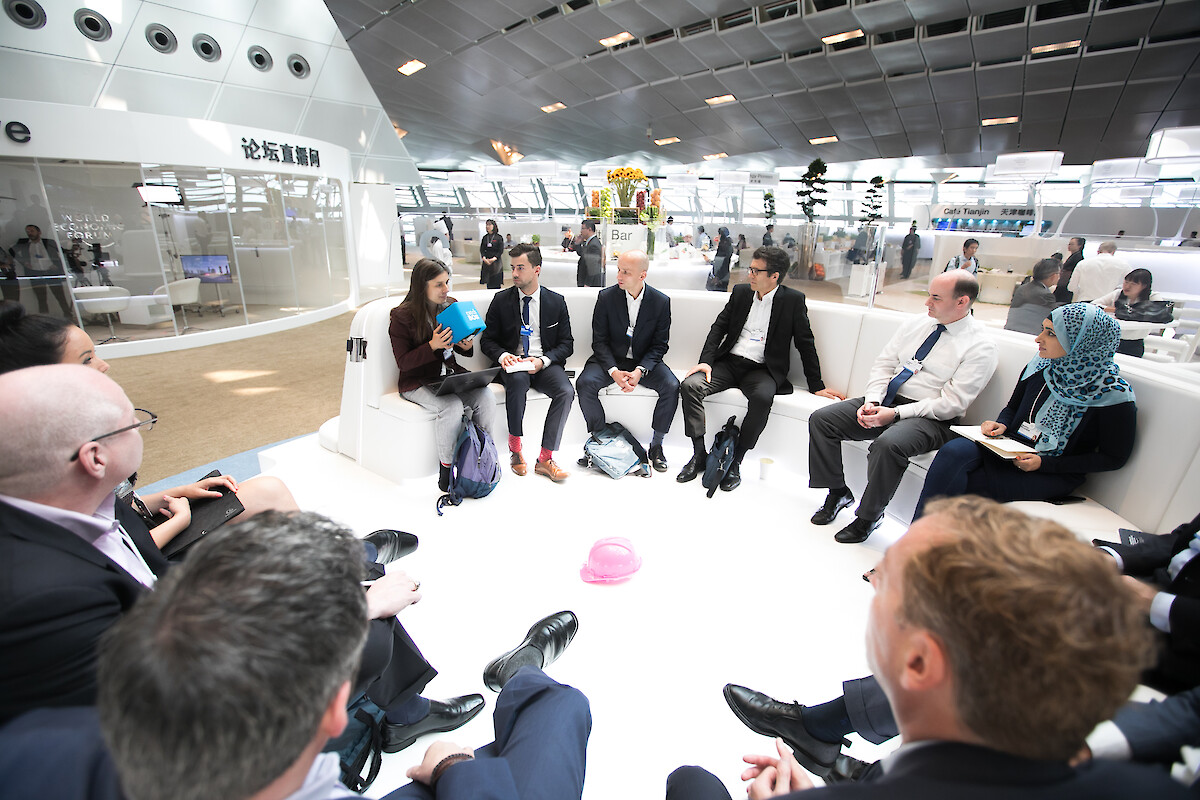
To reflect on this series of guest lecturers on social-ecological perspectives, I think my perspective as a physical geographer has been challenged for good. The social aspect of viewing environmental issues is not only important but also fundamental. To combat climate change and achieve sustainable development, interdisciplinary dialogues are the stepping stone, which can be followed by collaborative research and technical implementations. As a physical geographer who studies remote sensing and cartography, I think my background can contribute to such dialogue/research through ways such as helping to reconstruct baseline environmental conditions for assessing conservation efforts, quantifying carbon sequestration potential of forest restoration efforts, and implementing web-based maps that help connect research results with practitioners. I know all of you come from unique backgrounds, and I invite you to think of ways how your background contributes to this dialogue, so let's talk!
Reference:
Adger, W. N. (2000). Social and ecological resilience: Are they related? Progress in Human Geography, 24(3), 347-364. https://doi.org/10.1191/030913200701540465
Folke, C. (2006). Resilience: The emergence of a perspective for social-ecological systems analyses. Global Environmental Change, 16(3), 253-267. https://doi.org/10.1016/j.gloenvcha.2006.04.002
Holling, C., Schindler, D., Walker, B., & Roughgarden, J. (1995). Biodiversity in the functioning of ecosystems: An ecological synthesis. In C. Perrings, K. Maler, C. Folke, C. Holling, & B. Jansson (Eds.), Biodiversity Loss: Economic and Ecological Issues (pp. 44-83). Cambridge: Cambridge University Press. doi:10.1017/CBO9781139174329.005
Holling, C.S., 1996. Engineering resilience versus ecological resilience. In:
Schulze, P. (Ed.), Engineering Within Ecological Constraints. National Academy Press, Washington DC, pp. 31-44.
Science benefits from diversity. (2018). In Nature (Vol. 558, Issue 7708, p. 5). NLM (Medline). https://doi.org/10.1038/d41586-018-05326-3
Raworth, K. (2012). A Safe and Just Space for Humanity: Can we live within the doughnut? Oxfam Discussion Papers.
Tracking The Performance - Sustainable Agriculture Matrix. (2020). Retrieved September 27, 2020, from http://research.al.umces.edu/sam/trackingtheperformance/