The Shellfish Gene
Kate Petersen ·Oyster populations in the Northeastern United States have reached historic lows as a function of overharvesting, disease, and habitat degradation. Considered a keystone species, oysters provide critical ecosystem services. They clean the water, allowing sunlight to reach underwater plants. They support wildlife as food and shelter. Oyster reefs moderate wave action and prevent shoreline erosion. The oyster fishery is economically important and represents a way of life for generations of Northeastern residents.
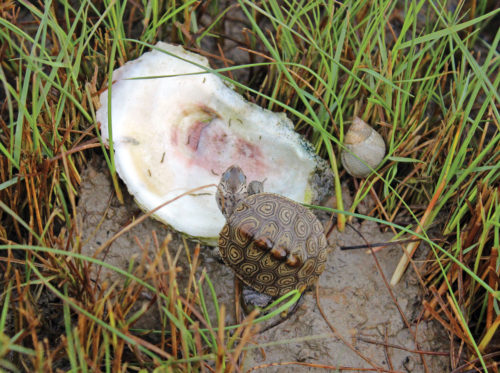
Could Hatchery Breeding Impact Restoration Efforts?
Active and coordinated restoration efforts include re-seeding Northeast estuaries and shorelines with oyster “spat,” larval oysters growing on a small surface. In the Chesapeake Bay, restoration managers typically use spat derived from wild broodstock and rotate plantings to maintain as much genetic diversity as possible. However, in regions of the Northeast where oysters are functionally extinct, spat are often bred from aquaculture lines, which have been subject to hatchery environmental conditions for multiple generations. Dr. Katherine McFarland and her colleagues at the University of Maryland Center for Environmental Science are investigating whether these different conditions cause genetic changes in farm-raised oysters that could result in reduced fitness when introduced to the wild. This phenomenon has been documented in captive-bred fish populations and could complicate restoration efforts.
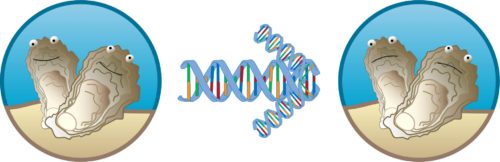
There are a few ways that hatchery conditions could drive genetic changes in a population. The first is through a “bottleneck effect,” wherein the genetic diversity of a new population is limited by the genetic diversity of the founder population. In this case, a relatively small number of wild oysters were, at one point, taken from the Bay and brought to the hatchery, limiting potential genetic variability to that present in the original group. Second, altered conditions in the hatchery could select for oysters best suited to those conditions. Oysters that could not survive in the new conditions would die, further limiting diversity. A third factor, specific to commercial aquaculture conditions, is intentional selection by hatchery managers of oysters most desirable for market viability, oysters that grow larger faster, those that are resistant to hatchery diseases, etc.
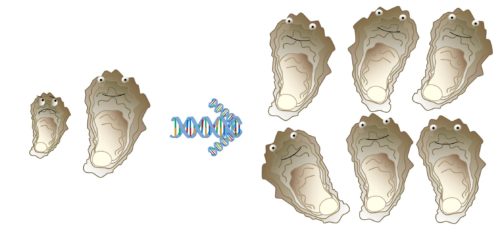
Because hatcheries tend to have uniform and predictable feeding schedules during larval rearing, whereas wild ecosystems are subject to more dynamic nutrient flows, Dr. McFarland chose to first test responses to food variability in oyster larvae produced from wild and aquaculture broodstock. As is often the case in a preliminary investigation, her team’s results do not conclusively confirm or disprove the potential of reduced fitness in farmed oysters. Instead, they help clarify the best direction of further inquiry. Dr. McFarland describes her results as “… very preliminary … right now, the results are … interesting from an energetics perspective more than a comparison between lines.”
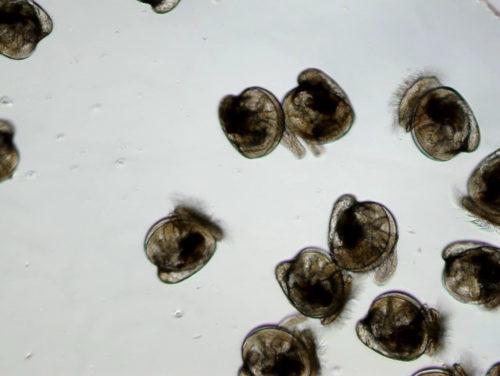
RESULTS
The first important finding was that, for all oyster lineages, growth stopped or slowed during starvation, but resumed once food became available. However, growth was not found to be compensatory. That is, the larvae did not accelerate growth in order to achieve metamorphosis within a standard time frame. They just resumed growth at a normal rate and went through metamorphosis later. While growth was not accelerated, respiration rates showed a spike of activity at a relatively consistent time interval after feeding resumed. This increased metabolic activity returned to baseline shortly thereafter, suggesting that the larvae may utilize a temporary period of elevated metabolic activity to reorganize biochemically or repair tissue loss or damage.
Although farmed and wild oyster larvae had similar growth rates following starvation, farmed month-old spat had higher baseline metabolic rates than wild spat when tested at multiple salinities. Differences in metabolic process between lines could be a very relevant finding for restoration managers. However, good science demands that this finding be thoroughly investigated before any conclusions are drawn. It is completely possible, for instance, that this difference will not have any ramifications for survival or that it foretells a big growth spurt for the farmed line. Determining the significance of this finding will require much more research.
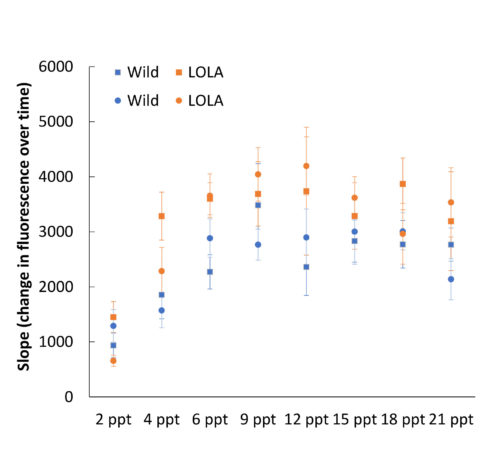
RNA SEQUENCING
Physiological responses are a highly relevant, but indirect, way to look at differences in genetic expression or makeup. Another way is to measure gene expression levels in the study organism through RNA sequencing. RNA levels tell researchers how much certain genes are being expressed in an organism. While an organism’s genetic code does not change, genetic expression changes all the time, as part of normal diurnal cycling, or in response to stress events. In this case, RNA samples were taken before, during, and after food deprivation events for both wild and farmed oysters, to determine which genes were active and to what degree. Results TBA!
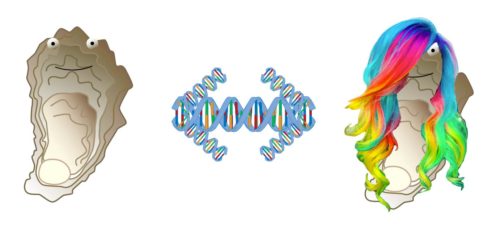
Dr. McFarland’s work with oyster physiology and genetics highlights the complex set of relationships between genes and organisms, and organisms and ecosystems. It also bespeaks the challenges inherent in human attempts to restore degraded ecological systems. Coastal Northeasterners owe a debt of gratitude to Dr. McFarland and the other restoration minded scientists who, through diligent, collective effort, help rehabilitate the ecosystems upon which we all depend.
To read about Dr. McFarland’s past work with oysters in New York City, check out: "Restoring oysters to urban estuaries: redefining habitat quality for eastern oyster performance near New York City."